Favorite Articles of the Moment
Disclaimer
• Your life and health are your own responsibility.
• Your decisions to act (or not act) based on information or advice anyone provides you—including me—are your own responsibility.
Recent Articles
-
We Win! TIME Magazine Officially Recants (“Eat Butter…Don’t Blame Fat”), And Quotes Me
-
What Is Hunger, and Why Are We Hungry?
J. Stanton’s AHS 2012 Presentation, Including Slides
-
What Is Metabolic Flexibility, and Why Is It Important? J. Stanton’s AHS 2013 Presentation, Including Slides
-
Intermittent Fasting Matters (Sometimes): There Is No Such Thing As A “Calorie” To Your Body, Part VIII
-
Will You Go On A Diet, or Will You Change Your Life?
-
Carbohydrates Matter, At Least At The Low End (There Is No Such Thing As A “Calorie” To Your Body, Part VII)
-
Interview: J. Stanton on the LLVLC show with Jimmy Moore
-
Calorie Cage Match! Sugar (Sucrose) Vs. Protein And Honey (There Is No Such Thing As A “Calorie”, Part VI)
-
Book Review: “The Paleo Manifesto,” by John Durant
-
My AHS 2013 Bibliography Is Online (and, Why You Should Buy An Exercise Physiology Textbook)
-
Can You Really Count Calories? (Part V of “There Is No Such Thing As A Calorie”)
-
Protein Matters: Yet More Peer-Reviewed Evidence That There Is No Such Thing As A “Calorie” To Your Body (Part IV)
-
More Peer-Reviewed Evidence That There Is No Such Thing As A “Calorie” To Your Body
(Part III)
-
The Calorie Paradox: Did Four Rice Chex Make America Fat? (Part II of “There Is No Such Thing As A Calorie”)
-
Interview: J. Stanton on the “Everyday Paleo Life and Fitness” Podcast with Jason Seib
|
As you might have heard, nearly all of the AHS 2013 videos are unwatchable due to technical and production failures. Therefore, I’m publishing my own presentation here in written form, exactly as it was given at the 2013 Ancestral Health Symposium in Atlanta—including slides.
This work is likely to be controversial, as it directly contradicts a great deal of received wisdom—both within mainstream obesity research and within the ancestral health community. The evidence places the energy requirements of the individual cell, and defects of energy production at the cellular level, at the beginning of the causal chain of both obesity and the metabolic syndrome.
However, as my bibliography shows, both metabolic flexibility and its origin in mitochondrial energy production are well-established, easily measurable phenomena—particularly within the field of exercise physiology—and the body of research continues to accumulate, finding defects of cellular energy production within the pathology of numerous diseases, from Alzheimer’s to Type II diabetes. It’s an exciting field—and it gives us both a theoretical framework in which to understand real-world phenomena, and many useful takeways for everyday life.
Yes, these pathologies are multifactorial. And, as I said in my 2012 AHS presentation on hunger, I don’t claim to have made a revolutionary new discovery. I’m bringing an existing field of research to light, and integrating it into our understanding.
So: here it is. Put on your thinking cap and enjoy.
Introduction
Hello. I’m J. Stanton, gnolls.org.
 You may wish to open the bibliography in another browser tab or window so that you can follow the references.
Life requires energy.
The human body contains somewhere between 10 and 100 trillion individual cells. Each one of these trillions of cells must continually convert fuel into energy in order to keep itself alive—and, thereby, keep us alive. In fact, a substantial fraction of our body mass is dedicated to providing a constant supply of fuel to every one of our trillions of cells, and removing the waste products of their energy production. Our respiratory system; our digestive system; our circulatory and lymphatic systems; substantial parts of our endocrine and nervous systems; and, lest we forget, each of these systems is itself made up of cells with their own energy requirements.
In short, the reason that large animals, including humans, are so heinously complicated is because every one of our 50-plus trillion cells requires a massive, complex, interdependent infrastructure to ensure it receives both an uninterrupted supply of fuel and of the cofactors required to burn it—independent of wild variances in energy demand via activity level and external temperature, and wild variances in energy supply via meal timing and composition.
Therefore, when we’re asking high-level questions such as “What causes obesity and the metabolic syndrome? And why did that process accelerate so dramatically after 1979?” it behooves us to examine our internal infrastructure—our energy supply chain—for bottlenecks and disruptions.
(Please note: While I’m happy to throw around terms like homeostasis and oxidative phosphorylation, I will use layman’s terms when possible in order to make this talk more accessible.)
What Is Metabolic Flexibility?

Unlike an automobile engine, which usually requires one very specific type and composition of fuel, most of the cells in our body can burn several very different fuels. However, we produce the overwhelming majority of our energy from two of them: glucose, the sugar from which starch is also formed, and fat. Metabolic flexibility is the ability to switch back and forth between the two major energy substrates—glucose and fat—based on availability and need.
How Is Metabolic Flexibility Measured?
Without a steady supply of oxygen, our cells can only produce a small amount of energy; they can only produce it from glucose; and the waste products quickly build up within the cell and prevent further energy production. This is called anaerobic respiration: it’s why we can’t sprint or hold our breath for very long, and why lack of oxygen, via blood loss, heart failure, or stroke, kills us so quickly.
In contrast, our cells require oxygen to efficiently extract all the energy from glucose. Furthermore, they require oxygen to burn fat at all! So except for a few specialized tissues, like red blood cells, and a few temporary conditions, like heavy sprinting and holding our breath, almost all of our energy comes from burning fuel with oxygen—called aerobic respiration.

Now. It turns out that a molecule of glucose contains six oxygen atoms—whereas a fat molecule contains only one, and releases a far greater amount of energy to the cell when we burn it. So while fat contains more energy by weight, it takes more oxygen to extract that energy from fat than it does from carbohydrate.

Next: Oxygen enters our body as oxygen gas (O2), but once it’s been used to burn fuel, it leaves as CO2—carbon dioxide. So we can hook a person up to a respirator, and compare how much oxygen and carbon dioxide they inhale with how much they exhale. This process is called “indirect calorimetry”, and it produces two things. First, we get an approximation of how much energy a person is consuming. Second, it produces what is called the Respiratory Exchange Ratio, also called the Respiratory Quotient— an approximation of how much glucose vs. how much fat your body is burning for energy. The RER, or RQ, varies between 0.7, representing 100% fat oxidation, and 1.0, representing 100% glucose oxidation…so lower values mean we’re burning more fat, and higher values mean we’re burning more glucose.
It’s important to note that the RER, or RQ, is a somewhat blunt instrument: it doesn’t account for protein oxidation, gluconeogenesis, or anaerobic respiration, and rapid changes can throw it off. However, it’s a good approximation that is relatively easy and non-invasive to measure.
Why Is Metabolic Flexibility Important?
Both the availability of energy, and our usage of it, change dramatically over time. We can be sleeping or sprinting; cold or hot; eating or fasting; consuming meat, fish, vegetables, potatoes, popcorn, or a Big Gulp. Our ability to adapt to these conditions depends greatly on the ability of our individual cells to be metabolically flexible—particularly our muscle cells, which use the majority of energy from our bodies. For instance:
- When we ingest carbohydrate, metabolic flexibility helps us control blood glucose, by burning glucose instead of fat. Our RER should be high.
- When we ingest fat without carbohydrate, met flex helps us burn the fat instead of storing it. Our RER should be low.
- When we fast (and everyone “fasts” while they sleep), met flex helps us burn the fat stored on our butt, instead of becoming hungry for sugar or going catabolic. Our RER should be low.
- When we exercise, met flex lets us burn more stored fat and produce more energy at all levels of effort. We can run faster, jump higher, move more weight, and go longer on less food before we “hit the wall.”
What Happens When Metabolic Flexibility Is Impaired?

When met flex is impaired, our cells can’t easily switch fuel sources: the RER, or RQ, is stuck in the middle. This leaves us unable to respond well to changing conditions. For instance:
- When we ingest carbohydrate, we cannot dispose of blood glucose as quickly as we should. This causes poor glycemic control—wide blood sugar swings.

- Our ability to burn fat in response to high-fat meals is reduced.
- Consequently, we have a decreased ability to increase energy output after meals (called “post-prandial thermogenesis”.)

- When we fast, our ability to burn our own fat is diminished.
- Since our ability to burn fat is diminished, we have a continual demand for glucose, even at rest. Therefore, fasting makes us hungry more quickly—and becomes catabolic more quickly.
- Therefore, a metabolically inflexible person attempting to fast or restrict food intake is very likely to also reduce their metabolic rate.
- When we exercise, our ability to burn our own fat is diminished, and our demand for glucose increases.
What Are The Real-World Consequences Of Impaired Metabolic Flexibility?
Now that we know what’s happening internally, let’s examine what that means. In particular, I ask those with experience losing weight, and in attempting to maintain a weight-reduced state, to listen closely and critically.
- If we have poor glycemic control, that means we have higher and more rapid blood sugar spikes and crashes. We all know this is unhealthy—but it also makes us more dependent on stimulants and bready, sugary snacks to maintain our mood, our attention span, and our ability to stay awake after meals.
- A continual demand for glucose at rest means we will become hungry much sooner after eating. Specifically, we become hungry for sugar and carbohydrate in order to “keep our energy up”
- Then, if we manage to ignore these hunger signals through willpower, our body is likely to reduce our energy expenditure in response, making us feel tired and listless, and making weight loss even more laborious. (At extremes, this can manifest itself as poor cold tolerance, and other signs and symptoms of hypothyroidism.)
- Consequently, intermittent fasting and heavy caloric restriction are likely to be both difficult and unsuccessful for the metabolically inflexible. And I suspect this to be a primary mechanism behind the so-called “low carb flu”.
- Finally, a continual demand for glucose during exercise means we will be dependent on a steady supply of energy drinks, bars, gels, and other sugary treats in order to perform physical activities that usually don’t even burn the energy we’ve ingested.
Do these consequences sound familiar to anyone?
Why Is Metabolic Flexibility Impaired?
To answer this question, we must first ask “Is metabolic flexibility a byproduct of neural and endocrine signaling, or is it an intrinsic property of individual cells?”
It turns out that if we measure the metabolic flexibility of individuals, then remove a sample of their muscle tissue and measure its response to fat and glucose in vitro (i.e. outside its neural and endocrine environment), we find the following:
“The interindividual variability in metabolic phenotypes was preserved in human myotubes separated from their neuroendocrine environment, which supports the hypothesis that metabolic switching is an intrinsic property of skeletal muscle.” (Ukropcova 2005. Also see Corpeleijn 2010, Berggren 2008)
So: while elucidating the precise mechanisms involved is still what’s known as an “active research area,” we can be reasonably sure that we are not just looking at an artifact of a broken hypothalamus or a dysfunctional HPTA axis.
Metabolic inflexibility is a metabolic defect at the level of the individual cell, multiplied by the trillions of cells that make up our muscle tissue until the effects become directly and objectively measurable via the respiratory exchange ratio.
What is this defect? Actually, there are two.
Metabolic inflexibility towards glucose—a low “insulin-stimulated RER”, the inability to increase glucose oxidation—appears to be limited by the glucose disposal rate: cells can’t burn more glucose because they’re insulin resistant. They can’t absorb it quickly enough.
In contrast, metabolic inflexibility towards fat—the inability to increase fat oxidation in response to fasting or fat intake—appears to be caused by mitochondrial dysfunction. (Mitochondria are the tiny organelles within each of our cells that actually perform aerobic respiration, turning fuel and oxygen into energy via the citric acid cycle—also known as the Krebs cycle or the TCA cycle.)
And while I’ll save the citation bombardment for my bibliography, which is available online,
I’ll note that this is both a subject of extensive recent research and a robust experimental result. Sample quote:
“Upon a more thorough analysis of the different components of metabolic flexibility, we found that in vivo mitochondrial function was the single predictor of basal RER.” (van den Weijer 2013)
To summarize the current consensus:
- Impaired glucose oxidation, an inappropriately low RER, is due to insulin resistance.
- Impaired fat oxidation, an inappropriately high RER, is due to mitochondrial dysfunction.
- Both are metabolic defects at the level of the individual cell.
How Does Metabolic Flexibility Become Impaired?
This is a fascinating subject and a continuing area of active research, and I would require another presentation to do it justice—so permit me to summarize the current state of the scientific literature.
First, an important note: obesity and metabolic inflexibility are not equivalent! Significant populations exist of both obese normoglycemics and skinny Type 2 diabetics. That being said, let’s explore some possible causal relationships.
- Higher basal RER is associated with subsequent weight gain, independent of total energy expenditure. (Zurlo 1990)
- Fasting, post-prandial, and exercise-stimulated fat oxidation are impaired in the prediabetic. (Corpeleijn 2010)
- When overfed, healthy first-degree relatives of Type 2 diabetics gain substantially more weight than those without such a family history. (Jenkins 2013)
- Quote: “…An impaired ability to increase fatty acid oxidation precedes the development of insulin resistance in genetically susceptible individuals.” (Heilbronn 2007)
- Quote: “Metabolic inflexibility, lower adaptation to a HFD, and reduced muscle mitochondrial mass cluster together in subjects with a family history of diabetes, supporting the role of an intrinsic metabolic defect of skeletal muscle in the pathogenesis of insulin resistance.” (Ukropcova 2007)
In other words, fat oxidation seems to fail first—and though we don’t yet know how, insulin resistance appears to be among the consequences of it. And in support of the mitochondrial theory:
- Quote: “…Mitochondrial content is lower across the continuum of insulin sensitivity and is not limited to T2DM.” (Chomentowski 2011)
- Mitochondria from obese Type 2 diabetics oxidize fat at a lower rate, even after adjusting for mitochondrial mass.
- Mitochondrial dysfunction is observed in relatives of Type 2 diabetics that are not yet themselves insulin-resistant.
In summary:
- Impaired met flex begins with an inability to increase fat oxidation in response to fasting, diet, or exercise.
- This propensity is strongly heritable, probably due to mitochondrial dysfunction.
- Insulin resistance follows, which causes an inability to increase glucose oxidation in response to diet. We are now metabolically inflexible.
- Impaired metabolic flexibility is not a byproduct of a broken brain, a damaged liver, or a faulty thyroid. It is a pair of metabolic defects at the level of the individual cell, multiplied by the trillions of cells that make up our muscle tissue until the effects become directly and objectively measurable via the respiratory exchange ratio.
How Can We Regain Our Metabolic Flexibility?
First, we must remember that we have multiple problems: metabolic inflexibility towards glucose, which manifests itself as poor glycemic control—and metabolic inflexibility towards fat, which manifests itself as poor response to fasting, calorie restriction, and low-carb diets.
The proven way to improve your insulin sensitivity—and, thereby, your met flex towards glucose—is to decrease your fat mass. However, weight loss alone does not improve fat oxidation! Basal RER remains just as elevated in the post-obese as it does in type 2 diabetics and the pre-obese.
So, we have successfully restated the problem as “To lose fat, first you must lose fat.”
And now it’s time to talk about exercise. We know that exercise temporarily helps dispose of blood glucose even in the insulin resistant, and that insulin sensitivity temporarily increases after exercise. However, what’s far more important is that exercise, unlike weight loss, is proven to restore fat oxidation—both basal RER and in response to a high-fat challenge meal.
“…A defect in the ability to oxidize lipid in skeletal muscle is evident with obesity, which is corrected with exercise training but persists after weight loss.” (Berggren 2008)
So. How much exercise do we need? We don’t know the minimum amount…but 3-5 45-minute sessions of moderately intense aerobic exercise per week, or 3 30-minute moderate aerobic sessions and one session of weight training, have been tested and proven sufficient to restore basal rates of fat oxidation to the level of healthy control subjects. And if you’re willing to do that 30-45 minutes every day, you can do so in about ten days.
Let me be clear. Exercise is not important because it burns calories! Exercise without calorie restriction is a remarkably ineffective weight loss intervention, because it usually makes us hungry enough to replace the calories we burn. Exercise is important because it restores your ability to oxidize fat—both when fasting and after meals. And we can tie this in with mitochondrial dysfunction by noting that exercise is proven to increase mitochondrial volume.

Caveat: your results may vary. Here’s the response of several healthy, lean individuals to a decrease in carbohydrate content of their diet: all over the place. So just because a study says the average obese person restored their metabolic flexibility after ten days of consistent exercise doesn’t mean you will.
Other interventions: We can decrease the carbohydrate content of our diet. For someone in energy balance, over time, basal RER will tend to converge towards the ratio of carbohydrate to fat in the diet. However, contrary to dogma which says “Don’t exercise until you’re fat-adapted,” it might be better to start exercising before playing with macronutrients—so that you have the metabolic flexibility to use them.
Finally, since the metabolically inflexible will tend to go catabolic more quickly, it’s probably a good idea to ensure you’re eating plenty of good-quality protein with each meal. And, while continual snacking is counterproductive, it’s probably not a good idea to try intermittent fasting right away. In fact, tolerance for fasting is a reasonably good diagnostic for impaired fat oxidation: if you feel light-headed after a few hours without food, you may be experiencing metabolic inflexibility regardless of your weight, bodyfat percentage, or BMI.
Conclusions and Summary
- Metabolic flexibility is the ability of our bodies to switch back and forth between their two major energy substrates: glucose and fatty acids.
- Met flex allows us to control blood sugar after eating, burn fat while fasting, and otherwise respond appropriately to changes in energy supply and demand.
- Met flex is typically measured via changes in the Respiratory Exchange Ratio (RER), aka the Respiratory Quotient (RQ), which approximates the ratio of fat to carbohydrate our bodies are burning during the time period measured.
- Metabolic inflexibility begins as a cellular-level impairment of the ability to increase or diminish fat oxidation.
- Impaired fat oxidation contributes to insulin resistance, and a consequent inability to increase or diminish glucose oxidation.
- Current evidence suggests that impaired fat oxidation is mitochondrial in origin, genetically and epigenetically heritable, and is among the causes, rather than the consequences, of obesity and insulin resistance.
- Moderate exercise can restore your ability to oxidize fat. Fat loss can restore your ability to absorb and oxidize glucose.
Takeaways
- You can’t exercise your way out of a bad diet.
- You can’t diet your way out of not exercising.
- Exercise is not important because it burns calories: it’s important because it restores metabolic flexibility.
So:
- Eat plenty of complete protein.
- Play frequently.
- Push your limits occasionally.
And whether we brand it as “Paleo,” “Primal,” “Ancestral Health,” or, as I do, “Eat Like A Predator”—it’s good to know that our recommendations are on a sound footing—evolutionarily, empirically, and biochemically.
I’m J. Stanton, gnolls.org. Thank you.
(My bibliography is available at this link.)
I am confident that this avenue of research will continue to provide both explanations for real-world phenomena, and useful takeaways for everyday living. Much more fascinating data has come to light in the months since this presentation—and though we are in the early stages of integration, I currently believe pathologies as disparate as depression, Parkinson’s, and the metabolic syndrome will be linked via defects of energy production at the level of individual cells. Further, I believe that the resulting neural and hormonal imbalances will frequently be shown as effects, not causes: attempts by the brain (and other regulatory organs) to maintain a broken homeostasis.
Yes, these pathologies are multifactorial…and we’re still trying to figure out exactly how mitochondrial energy production becomes impaired. However, I wish to be clear about which direction the evidence is currently leading me.
Live in freedom, live in beauty.
JS
I’m proud of this one: spread it using the widgets below. Also, this is your last chance to get a “Die Biting The Throat” T-shirt—so jump on over to the pre-order page and pick one up before they go away.
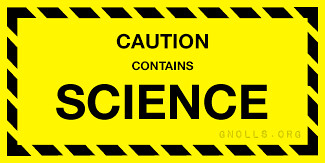
Important note! For a more up-to-date exploration of this subject, I strongly recommend my 2013 AHS presentation “What Is Metabolic Flexibility, And Why Is It Important?”
Most of us who eat a low-carbohydrate diet—Paleo, Primal, Atkins, or otherwise—experience anywhere from a couple days to a couple weeks of low energy as we adjust to it, an experience known informally as the “low carb flu”. And some people never seem to adjust.
Here’s why—and here are some ideas that might help you if you’re having trouble adjusting!
Note that low-carb isn’t an objective of a paleo diet: it’s just the usual consequence of eliminating grains and sugars.
It’s certainly possible to eat a higher-carb paleo diet—and it’s a good idea if you’re doing frequent, intense workouts like HIIT, Crossfit, or team sports after school—but you’d have to eat a lot of potatoes and bananas to get anywhere near the same amount of carbohydrate you used to get from bread, pasta, cereal, and soda.
Burning Food For Energy: Glycolysis and Beta-Oxidation
Our bodies have several ways to turn stored or ingested energy into the metabolic energy required to move around and stay alive. This is called cellular respiration.
The two main types of cellular respiration are anaerobic (which does not require oxygen) and aerobic (which requires oxygen). Anaerobic metabolism, also known as fermentation, is nineteen times less efficient—and we can only maintain it for short periods, because its waste products build up very quickly. This is why we can’t sprint for long distances.
We spend most of our time in aerobic metabolism. Our two primary aerobic sources of energy are glycolysis, which converts glucose to energy, and beta-oxidation, which converts fat to energy.
A Short Metabolic Digression Explaining The Above (Optional)
Strictly speaking, glycolysis is the start of both the aerobic and anaerobic oxidation of glucose: it converts glucose to pyruvate.
In the aerobic oxidation of glucose, the pyruvate is transported into the mitochondria, whereupon it is converted to acetyl-CoA and fed into the TCA cycle (aka the citric acid cycle or Krebs cycle, depending on how long ago you took your biology course.) This produces 19 times more energy than the original glycolysis!
In the anaerobic oxidation of glucose (“lactic acid fermentation”), the pyruvate is instead converted to lactic acid, which produces no more energy.
In the aerobic oxidation of fat (“beta-oxidation”), the fat is transported into the mitochondria, whereupon it is sliced up into individual acetyl-CoA molecules, each of which enters the TCA cycle.
Humans have no way to anaerobically oxidize fat.
 A graphic flowchart of glycolysis. You know it's SCIENCE! because there are a lot of molecules and arrows.  And here's a flowchart of beta-oxidation.
Glucose is the simple sugar all cells use for glycolysis, and it’s the most common. The other simple sugars we can digest are galactose (found primarily in milk), which we convert to glucose—and fructose (found primarily in fruit, table sugar, corn syrup, and honey), which our liver converts directly to glycogen or fat.
Starch is just a bunch of glucose molecules stuck together. In fact, “complex carbohydrates” in general are just sugars stuck together…and we can only absorb them through the intestine once they are broken down into individual simple sugars by our digestive system. In other words, all “carbohydrates” are just sugar.
There is a lot more to talk about here, including glycogen storage and retrieval, gluconeogenesis, and de novo lipogenesis…but explaining all the pathways of digestion, energy storage, and cellular respiration would be an entire book in itself!
Moving on: while it’s OK for fat to hang around in our bloodstream for a while, high blood sugar is actively toxic to our tissues. (The long-term consequences of untreated diabetes—heart, kidney, nerve, eye, and muscle damage, leading to numbness, blindness, amputations, strokes, and death—are basically just long-term glucose poisoning.) So after we eat something containing any amount of carbohydrate, insulin ensures that the glucose is immediately taken into our cells and either burned for energy, stored, or converted into palmitic acid—a saturated fat!
This is why a “low-fat, high-carb” diet is really a high-fat diet. Unless your “high-carb” diet involves an intravenous glucose drip carefully metered to keep your blood sugar constant, most of the ‘carbohydrates’ (sugars) you eat will be converted either to glycogen or to palmitic acid (again, a saturated fat) before you use them. “Soluble fiber” and other indigestible carbohydrates are fermented into short-chain saturated fats, like butyric acid, in your colon. Fructose, of course, is converted directly to liver glycogen or to palmitic acid. And if you’re losing weight by burning your own fat, keep in mind that human fat has roughly the same composition as lard—approximately 40% saturated!
You might ask yourself if it makes sense that natural selection would select us to store energy in the form of something directly harmful to us. If saturated fat is really so terrible, and polyunsaturated fat is really so healthy, why doesn’t our body store energy as linoleic acid, like grains do?
Metabolic Flexibility and the Respiratory Exchange Ratio
If we have excess glucose in our bloodstream, our muscles will burn it first, because it’s toxic. But eventually we run out of glucose, and that’s when our bodies need to switch over to beta-oxidation—burning fat. The ability to switch back and forth between the two processes is called “metabolic flexibility” in the scientific literature.
Metabolic flexibility varies dramatically from individual to individual, which we would expect based on the widely varying experiences people report with low-carb diets. So how do scientists figure out what fuel our bodies are burning?
It turns out that beta-oxidation (fat-burning) produces less carbon dioxide than glycolysis (sugar-burning)—and we can measure that in our breath. The ratio of CO2 to O2 is 0.7 for beta-oxidation and 1.0 for glycolysis…so an RER (Respiratory Exchange Ratio) of 0.7 indicates pure fat-burning, and 1.0 and above indicates pure sugar burning. (You can read more about the RER here.)
Typical healthy people have a resting, fasting RER of approximately 0.8. Therefore, we can easily see that the frequent vegetarian and vegan claims of “Nothing else can provide any energy without first being converted to carbs” and “You can get plenty of energy from fat, but you have to go into ketosis to do it” are—like most nutritional claims made by veg*ans—complete bunk.
Metabolic Flexibility: The “Low Carb Flu” Is Not Your Imagination
It shouldn’t be a surprise that the obese and diabetic tend to have higher resting RERs, and that higher RER is a significant predictor of future obesity. If our ability to burn fat for energy is impaired, we’re going to have a hard time losing weight, and we’ll become ravenously hungry when our blood sugar runs out no matter how much fat we have available to burn.
Is this sounding familiar to anyone?
Sounds like the “low carb flu”, doesn’t it? When we talk about our metabolic “set point”, part of what we’re talking about is metabolic flexibility. It does no good to have a huge store of fat if we can’t burn it for energy!
Int J Obes Relat Metab Disord. 1992 Sep;16(9):667-74.
Fasting respiratory exchange ratio and resting metabolic rate as predictors of weight gain: the Baltimore Longitudinal Study on Aging.
Seidell JC, Muller DC, Sorkin JD, Andres R.
“…The adjusted relative risk of gaining 5 kg or more in initially non-obese men with a fasting RER of 0.85 or more was calculated to be 2.42 (95% confidence interval: 1.10-5.32) compared to men with a fasting RER less than 0.76.”
Furthermore, it turns out that people with a family history of type II diabetes, but who don’t yet have it themselves, have higher RERs and impaired metabolic flexibility.
Diabetes August 2007 vol. 56 no. 8 2046-2053
Impaired Fat Oxidation After a Single High-Fat Meal in Insulin-Sensitive Nondiabetic Individuals With a Family History of Type 2 Diabetes
Leonie K. Heilbronn1, Søren Gregersen2, Deepali Shirkhedkar1, Dachun Hu1, Lesley V. Campbell1
“…An impaired ability to increase fatty acid oxidation precedes the development of insulin resistance in genetically susceptible individuals.”
Also see:
AJP – Endo November 1990 vol. 259 no. 5 E650-E657
Low ratio of fat to carbohydrate oxidation as predictor of weight gain: study of 24-h RQ
F. Zurlo, S. Lillioja, A. Esposito-Del Puente, B. L. Nyomba, I. Raz, M. F. Saad, B. A. Swinburn, W. C. Knowler, C. Bogardus, and E. Ravussin
This is very important: we can see that impaired fat oxidation must be related to the causes, not the consequences, of obesity and diabetes. So we’ve struck another blow to “calories in, calories out”, and the idea that you’re fat just because you’re lazy.
In support of this theory, I note this paper, which contains the following graph of several individuals’ RER in response to a high-fat diet vs. a moderate-fat diet. Keep in mind that the change was only from 37% to 50% fat, which is relatively minor, and the paper doesn’t tell us what fats were being consumed…but this graph is still instructive:

The top graph is the average, the bottom graph is for each individual. Note that some adapted right away, some took several days, and some were still not adapted on day 4!
Higher RER isn’t all bad. It’s associated with having more fast-twitch muscle fibers, which are associated with a greater ability to build muscle mass. This fits the anecdotal evidence that people who gain fat easily also tend to gain muscle easily, whereas skinny people have a much harder time bulking up.
So maybe you’re lucky, or already in good health, and you adapt relatively quickly to a low-carb diet. But what if you’re not? What if you’re stuck with the “low carb flu”?
Regaining Your Metabolic Flexibility
Obviously we’d like to regain our metabolic flexibility. But how? Here’s one way:
Journal of Applied Physiology September 2008 vol. 105 no. 3 825-831
Separate and combined effects of exercise training and weight loss on exercise efficiency and substrate oxidation
Francesca Amati,1 John J. Dubé,2 Chris Shay,3 and Bret H. Goodpaster1,2
“…Exercise training, either alone or in combination with weight loss, increases both exercise efficiency and the utilization of fat during moderate physical activity in previously sedentary, obese older adults. Weight loss alone, however, significantly improves neither efficiency nor utilization of fat during exercise.”
Diabetes September 2003 vol. 52 no. 9 2191-2197
Enhanced Fat Oxidation Through Physical Activity Is Associated With Improvements in Insulin Sensitivity in Obesity
Bret H. Goodpaster, Andreas Katsiaras and David E. Kelley
“Rates of fat oxidation following an overnight fast increased (1.16 ± 0.06 to 1.36 ± 0.05 mg · min−1 · kg FFM−1; P < 0.05), and the proportion of energy derived from fat increased from 38 to 52%."
October 15, 2009 The Journal of Physiology, 587, 4949-4961.
Improved insulin sensitivity after weight loss and exercise training is mediated by a reduction in plasma fatty acid mobilization, not enhanced oxidative capacity
Simon Schenk1, Matthew P. Harber1, Cara R. Shrivastava1, Charles F. Burant1,2 and Jeffrey F. Horowitz1
“…Resting fatty acid oxidation was unchanged after the intervention in WL [weight loss]. Consistent with an increase in maximal oxidative capacity, resting whole-body fatty acid oxidation was increased more than 20% after WL + EX [weight loss + exercise].”
In other words, despite the title, weight loss plus exercise increased resting fat oxidation…but just losing weight did not!
AJP – Endo April 2008 vol. 294 no. 4 E726-E732
Skeletal muscle lipid oxidation and obesity: influence of weight loss and exercise
Jason R. Berggren,1,2 Kristen E. Boyle,1,2 William H. Chapman,4 and Joseph A. Houmard1,2,3
“10 consecutive days of exercise training increased (P ≤ 0.05) FAO [fatty acid oxidation] in the skeletal muscle of lean (+1.7-fold), obese (+1.8-fold), and previously extremely obese subjects after weight loss (+2.6-fold)…These data indicate that a defect in the ability to oxidize lipid in skeletal muscle is evident with obesity, which is corrected with exercise training but persists after weight loss.”
How about that? It turns out that exercise is important after all…not because of the calories you burn by exercising, which you usually replace right away because you’re hungry, but because it helps you regain metabolic flexibility. Exercise stimulates your body to burn more fat, both during exercise and at rest.
And that’s what health is about: we’re not interested in losing weight if it just means losing muscle. We’re interested in losing fat.
There are other benefits beyond fat loss, too: exercise tends to normalize broken metabolisms.
Diabetes March 2010 vol. 59 no. 3 572-579
Restoration of Muscle Mitochondrial Function and Metabolic Flexibility in Type 2 Diabetes by Exercise Training Is Paralleled by Increased Myocellular Fat Storage and Improved Insulin Sensitivity
Ruth C.R. Meex1, Vera B. Schrauwen-Hinderling2,3, Esther Moonen-Kornips1,2, Gert Schaart1, Marco Mensink4, Esther Phielix2, Tineke van de Weijer2, Jean-Pierre Sels5, Patrick Schrauwen2 and Matthijs K.C. Hesselink1
“Mitochondrial function was lower in type 2 diabetic compared with control subjects (P = 0.03), improved by training in control subjects (28% increase; P = 0.02), and restored to control values in type 2 diabetic subjects (48% increase; P < 0.01). Insulin sensitivity tended to improve in control subjects (delta Rd 8% increase; P = 0.08) and improved significantly in type 2 diabetic subjects (delta Rd 63% increase; P < 0.01). Suppression of insulin-stimulated endogenous glucose production improved in both groups (−64%; P < 0.01 in control subjects and −52% in diabetic subjects; P < 0.01). After training, metabolic flexibility in type 2 diabetic subjects was restored (delta respiratory exchange ratio 63% increase; P = 0.01) but was unchanged in control subjects (delta respiratory exchange ratio 7% increase; P = 0.22).”
Did you catch that? “Metabolic flexibility in type 2 diabetic subjects was restored”?
Unfortunately, this study didn’t measure resting fat oxidation, like the others—but it does suggest that there’s no need to kill yourself with “Biggest Loser”-style misery. 30 minutes of cycling at 55% of maximum effort twice a week, and one session of weight training once a week, was enough to restore metabolic flexibility. That doesn’t sound very intimidating, does it? (And there are many better and more entertaining ways to get half an hour of moderate aerobic exercise than sitting on a stationary bike.)
“Aerobic exercise was carried out on a cycling ergometer twice a week for 30 min at 55% of a previously determined maximal work load (Wmax). Resistance exercise was performed once a week and comprised one series of eight repetitions at 55% of subjects’ previously determined maximal voluntary contraction (MVC) and two series of eight repetitions at 75% MVC and focused on large muscle groups (Chest press, leg extension, lat pull down, leg press, triceps curls, biceps curls, abdominal crunches, and horizontal row).”
…Yet You Must Take Advantage Of Your Newfound Metabolic Flexibility
Of course, our newly-regained flexibility won’t help if we stuff ourselves with the government-recommended 7-11 servings of “heart-healthy whole grains” (= “carbs”, = sugar) per day, because we will be constantly burning sugar. Only when we’re done burning glucose can we use our newfound flexibility to burn some fat.
That’s one reason, among many, why I eat a paleo diet—and why I don’t snack. (For more on that subject, read “Why Snacking Makes You Weak, Not Just Fat”.)
A Short Digression: Please Stay Off The “Faileo Diet”
Some ‘paleo’ books still insist that saturated fat is bad for you and paleolithic people didn’t eat much of it, which is absolute nonsense. But your calories have to come from somewhere…if not fat, then from protein or carbohydrates. And since those same books also usually disallow potatoes and other convenient sources of starch, you’re basically stuck eating lots of lean protein.
As a result, you’ll eat very few calories, because of the satiating effect of protein—which is fine if you’re just trying to lose weight, but disastrous if you’re physically active, because you’ll be perpetually exhausted. This is why fat-phobic ‘paleo’ is sometimes called the “Faileo Diet”.
The Difference Between Beta-Oxidation and Ketosis
Here’s where I say something that might be controversial: I think going cold-turkey VLC (very low carb) or zero-carb makes the transition much harder, particularly for people who are already physically active.
Beta-oxidation (fat-burning) occurs nearly continually, and produces much of our energy at rest once insulin has cleared any sugar spike out of our system. However, our body does have some requirement for glucose, which it satisfies in the short-term primarily by having the liver make it—a process called gluconeogenesis.
If we eat zero carbs, or very few, over a period of time, our body enters a state called ketosis, in which some of our tissues that used to require glucose shift over to burning ketone bodies, which are alternative products of fat metabolism. And while it is true that our brains and hearts actually run more efficiently on ketones, it takes several weeks for our bodies to fully adapt. Meanwhile, we lack energy for high-effort activities, because our muscles are depleted of glycogen, which is made from glucose.
So you might not have the “low-carb flu”—you might be stuck in an unnecessary multi-week rut of keto-adaptation.
Interested in learning more about ketosis? Read Stephen Phinney’s “Ketogenic Diets and Physical Performance” (Nut Metab 2004, 1:2) for more information about ketosis and the process of keto-adaptation. Once you’ve read that, if you’re deeply interested in the ketotic state, try ketotic.org…and if you’re interested in a ketogenic diet that isn’t nutrient-deficient or disgusting, try these articles from the Drs. Jaminet (Ketogenic Diets I, Ketogenic Diets II).
There is a persistent myth that ketosis is dangerous: it’s not. People (including some doctors) commonly confuse it with ketoacidosis, a pathological state usually only found in uncontrolled diabetics and (rarely) raging alcoholics.
Even worse, you might be stuck in the state informally known as “Low Carb Limbo”—in which you’re eating too few carbohydrates to fuel high-effort, glycolytic activity, but too many carbohydrates to ever keto-adapt.
If you’re active and determined to keto-adapt, read this excellent article from Primal North, “Keto-Adaptation vs. Low Carb Limbo”.
Conclusion: Stay Out Of The Muddy Middle
In summary, it’s much easier and quicker to burn fat via beta-oxidation than it is to adapt to ketosis…so unless ketosis is your goal, you might be making your transition to a healthy diet much harder by keeping your carb intake too low.
I think that if we keep our carbohydrate intake near our body’s requirement while not in ketosis, which is perhaps 15-20% of total calories—and only eat those carbohydrates with meals involving complete protein and fat, not by themselves—most of us should be able to gain the fat-burning benefits of metabolic flexibility without suffering the pain of trying to adapt to ketosis. So if you’re new to Paleo or low-carb eating, you’re stuck with long-term “low-carb flu”, and especially if you’re already physically active, try adding some root starches like potatoes or sweet potatoes to your meals. (Or white rice, if you’re following the Perfect Health Diet.)
(And if you’re determined to keto-adapt, go fully ketogenic, as per “Keto-Adaptation vs. Low Carb Limbo.”)
Live in freedom, live in beauty.
JS
For more information, continue reading my 2013 AHS presentation “What Is Metabolic Flexibility, And Why Is It Important?”
Was this article helpful to you? Please share it using the buttons below.
|
“Funny, provocative, entertaining, fun, insightful.”
“Compare it to the great works of anthropologists Jane Goodall and Jared Diamond to see its true importance.”
“Like an epiphany from a deep meditative experience.”
“An easy and fun read...difficult to put down...This book will make you think, question, think more, and question again.”
“One of the most joyous books ever...So full of energy, vigor, and fun writing that I was completely lost in the entertainment of it all.”
“The short review is this - Just read it.”
Still not convinced?
Read the first 20 pages,
or more glowing reviews.
Support gnolls.org by making your Amazon.com purchases through this affiliate link:

It costs you nothing, and I get a small spiff. Thanks! -JS
.
Subscribe to Posts
|
Gnolls In Your Inbox!
Sign up for the sporadic yet informative gnolls.org newsletter. Since I don't update every day, this is a great way to keep abreast of important content. (Your email will not be sold or shared.)
IMPORTANT! If you do not receive a confirmation email, check your spam folder.
|