Favorite Articles of the Moment
Disclaimer
• Your life and health are your own responsibility.
• Your decisions to act (or not act) based on information or advice anyone provides you—including me—are your own responsibility.
Recent Articles
-
We Win! TIME Magazine Officially Recants (“Eat Butter…Don’t Blame Fat”), And Quotes Me
-
What Is Hunger, and Why Are We Hungry?
J. Stanton’s AHS 2012 Presentation, Including Slides
-
What Is Metabolic Flexibility, and Why Is It Important? J. Stanton’s AHS 2013 Presentation, Including Slides
-
Intermittent Fasting Matters (Sometimes): There Is No Such Thing As A “Calorie” To Your Body, Part VIII
-
Will You Go On A Diet, or Will You Change Your Life?
-
Carbohydrates Matter, At Least At The Low End (There Is No Such Thing As A “Calorie” To Your Body, Part VII)
-
Interview: J. Stanton on the LLVLC show with Jimmy Moore
-
Calorie Cage Match! Sugar (Sucrose) Vs. Protein And Honey (There Is No Such Thing As A “Calorie”, Part VI)
-
Book Review: “The Paleo Manifesto,” by John Durant
-
My AHS 2013 Bibliography Is Online (and, Why You Should Buy An Exercise Physiology Textbook)
-
Can You Really Count Calories? (Part V of “There Is No Such Thing As A Calorie”)
-
Protein Matters: Yet More Peer-Reviewed Evidence That There Is No Such Thing As A “Calorie” To Your Body (Part IV)
-
More Peer-Reviewed Evidence That There Is No Such Thing As A “Calorie” To Your Body
(Part III)
-
The Calorie Paradox: Did Four Rice Chex Make America Fat? (Part II of “There Is No Such Thing As A Calorie”)
-
Interview: J. Stanton on the “Everyday Paleo Life and Fitness” Podcast with Jason Seib
|
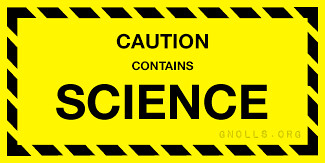
(Part IV of a series. Go back to Part I, Part II, or Part III, or skip to Part V.)
This is a long and detailed article, but it’s very important. I believe the conclusions justify the length: we’re done laying groundwork, and we’re finally starting to build some answers to the original question: “Why are we hungry?”
I must emphasize that I have no stake in any of the current controversies. I have no diet books for sale and no research thesis to defend, and I began this series long before the AHS. My concern is (as always) to organize and present the facts as I understand them to you, my readers, so you can draw useful conclusions about your own diet and life.
Furthermore, my diet at the moment contains roughly a Perfect Health Diet-compliant 15% of carbohydrate, plus whatever I need for intense physical activity (though I don’t count or track my intake), so I don’t believe I belong to either the high-carb or low-carb camps.
In previous installments, we’ve established the following:
- Hunger is not a singular motivation: it is the interaction of several different clinically measurable, provably distinct mental and physical processes.
- In a properly functioning human animal, likes and wants coincide; satiation is an accurate predictor of satiety; and the combination of hunger signals (likes and wants) and satisfaction signals (satiation and satiety) results in energy and nutrient balance at a healthy weight and body composition.
- Restrained eating requires the exercise of willpower to override likes, wants, and the lack of satiation or satiety; the exercise of willpower uses energy and causes stress; and stress makes you eat more. Therefore, a successful diet must minimize the role of willpower.
Now we can examine some of the ways that our hunger signals fail us.
It is important to remember that, by definition, all our hunger drives are in balance with our willpower at any moment in time! Otherwise we would be eating more or less than we are. The issue is that for many of us, this balance is only reached at an unhealthy weight or body composition—or it involves an excessively stressful amount of willpower. Part III explores this subject in detail.
Why Are We Ever Sated?
The desired result of eating is satiety: our body’s signal that it is replete with nutrients. But first, let’s ask a question: why are we ever sated? Since starvation is an animal’s primary concern, why didn’t Paleolithic humans simply eat themselves into obesity whenever possible?
We all know what happens if we eat a big meal just before intense exercise: at best, our performance suffers greatly, and at worst, we vomit. This is because digestion requires a meaningful amount of energy. Clearly it would be counterproductive to go hunting when our mental and physical performance is greatly impaired. Even foraging would be impaired, as gathering in the wild requires a keen eye and close attention, and the brain uses perhaps 20% of our energy at rest.
So it’s clear that we must wait until our body has either used or stored the energy and nutrients from our previous meal before we perform at our best. The fact that ghrelin is neurotrophic makes this clear: our brains only kick into high gear when it would be both possible and beneficial to acquire more food.
This suggests an obvious consequence: energy retrieval from storage is an important part of satiety. We don’t eat via a constant intravenous infusion of exactly the nutrients we need, at exactly the rate we are using them: we digest them and store them for use as needed. I’ll explore this in detail below.
 Not so well suited to the African savanna. A secondary motivation is that stored fat both traps heat and slows us down. Since humans evolved as diurnal (daytime-active) hunters and foragers on the African savanna, heat dissipation was often our limiting factor in procuring food. (This is most likely why we are hairless and have sweat glands, as opposed to the inches-thick layer of fat surrounding, say, a polar bear.) And, of course, fat adds weight: consider how much slower you’d run with an extra 20 pounds attached to you. (Put on some two-pound wrist and ankle weights, a vest with twelve pounds of sand in the pockets, and see how fast you run the 400-meter.) So while some fat accumulation would have been beneficial as a buffer against bad times, there was clearly a point beyond which fat accumulation would have impaired our ability to hunt and forage.
Everything Flows Downhill From Satiety
As we previously established, satiety is our body’s signal that it is replete with nutrients. Therefore, it should be obvious that nothing can make up for a lack of satiety: no amount of tricks to achieve temporary satiation will make up for a nutritional deficiency.
Satiety Is Not Generic
As stated back in Part II, “hunger” is not a generic drive, satisfiable by a generic substance called “food”. A properly functioning animal is hungry for different foods, depending on its nutritional status: even butterfiles—insects!—are smart enough to lick water off of mineralized rocks, and every animal, from aardvark to zebra, is capable of finding and ingesting the myriad nutrients it needs to survive.
The Salt-Mining Elephants of Kitum Cave
There are caves in Mount Elgon National Park, Kenya, partway up the shield of the extinct volcano Mount Elgon. (The best-known is called Kitum Cave.) The soil in the park is of recent volcanic origin, and the high rainfall washes away many of its minerals, leaving animals throughout the reserve deficient in many things—most critically, sodium.
Kitum Cave reaches over 700 feet into the side of Mount Elgon, and the cave complex of which it is a part contains the only known salt deposits in the region. Consequently, nearly every herbivore in the park must make periodic pilgrimages to the cave—up the side of the mountain, and over a long, narrow, dangerous path with no escape—in order to lick sodium sulfate from the rocks of Kitum Cave. In fact, it is plausibly argued that Kitum Cave was primarily created by the mining actions of elephants scraping salt from its walls and floor!
Joyce Lundberg and Donald A. McFarlane
Speleogenesis of the Mount Elgon elephant caves, Kenya
GSA Special Papers 2006, v. 404, p. 51-63
(fulltext, includes pictures and figures)
From Lundberg and McFarlane’s overview article, “Mount Elgon’s Elephant Caves”:
“The caves, of which Kitum and Makingeny are the best known, have long been known to attract elephants and other animals. The herbivores enter the dark cave interiors to consume salts, mainly mirabalite and sodium sulphate (Glauber’s salt) that effloresce from the cave walls. The crystals are gouged out by elephant trunks and bushbuck teeth and licked off wall by buffalo.”
 Elephants in Kitum Cave.
BBC 2 once aired an amazing documentary called “Elephant Cave”, which shows just how dangerous the round-trip to and from the cave is. Unfortunately it’s not available to watch online, but the enterprising web searcher can probably find a torrent of it.
Note that Kitum Cave is not the only example of a salt ingestion cave, just the largest known:
Lundquist Charles A., Varnedoe Jr. William W.
Salt ingestion caves
International Journal of Speleology, vol 35, issue 1, pp. 13-18, 2006.
(Note: containts link to fulltext PDF)
In conclusion, even small, skittish herbivores like bushbucks have instinctive hunger drives of sufficient discernment to motivate them to make a dangerous pilgrimage up a volcano to obtain just one of the many nutrients—sodium—they need to live.
We should not expect any worse from the hunger drives of a properly functioning human animal.
How Satiety Fails
Now we are ready to dig into the meat of this essay.
Satiety can fail in three ways:
- We fail to ingest the energy and/or nutrients our body requires.
- We fail to absorb the energy and/or nutrients our body requires.
- We cannot retrieve the energy and/or nutrients our bodies have stored.
Satiety Failure #1: Failing To Eat The Real Food We Require
We know from experience that no matter how many calories worth of Skittles or Oreos we eat, we won’t satisfy our hunger. Our stomachs might be full to bursting—but as soon as we have room to digest it, we’ll be hungry again, because Skittles and Oreos don’t give us the nutrients we need to live. And as I’ve explained above, satiety is not generic: if we’re short on any one of the hundreds of nutrients our body needs, we’ll keep eating until we get it.
I’ve linked this study before (hat tip to Fat Fiction), but since it’s so illustrative, I’ll link it again:
Y Li, C Wang, K Zhu, R N Feng and C H Sun
Effects of multivitamin and mineral supplementation on adiposity, energy expenditure and lipid profiles in obese Chinese women
International Journal of Obesity (2010) 34, 1070–1077
“After 26 weeks, compared with the placebo group, the MMS group had significantly lower BW [body weight], BMI, FM [fat mass], TC and LDL-C, significantly higher REE [resting energy expenditure] and HDL-C, as well as a borderline significant trend of lower RQ [respiratory quotient] (P=0.053) and WC [waist circumference] (P=0.071). The calcium group also had significantly higher HDL-C and lower LDL-C levels compared with the placebo group.”
How much is “significant”? From the summary:
“…The multivitamin and mineral group lost an average of 3.6 kg (8 pounds) of body weight, compared to 0.9 kg (2 pounds) and 0.2 kg (0.44 pound) for the calcium and placebo groups, respectively.
Protein targeting is another very important issue (previously discussed here.) Our bodies have an absolute requirement for complete protein—but unlike carbohydrate or fat, we have no storage depots for it. So if we don’t get complete protein in our diet, we must disassemble our own tissues to get it. (Previously discussed here.)
“Protein” is just chains of amino acids. “Complete protein” is protein containing all the essential amino acids—the ones we must eat because our bodies can’t make them—roughly in the proportions our body needs them.
On the other hand, if we eat too much complete protein, our bodies have a limited capacity to convert it into glucose…so we tend to desire neither too much nor too little complete protein. For more on protein targeting, including links to the scientific literature, read Dr. Paul Jaminet’s excellent summary, “Protein, Satiety, and Body Composition.”
(This satiety mechanism can be extended to other essential nutrients—but this article is already far too long!)
In closing, I’ll note that a can of Pringles has the same number of calories as a dozen large hard-boiled eggs. Which will leave you sated hours later: 32 Pringles (300 calories), made of seed oil and potato slurry—or four hard-boiled eggs (300 calories), containing 12g of complete protein and a host of vitamins, minerals, and essential nutrients like choline and lutein?
Satiety Failure #2: Failing To Absorb Nutrients
It doesn’t matter if we eat real food if we can’t absorb its nutrients.
Unfortunately, covering the various gut malabsorptions and dysbioses, such as celiac, IBS, Crohn’s, and SIBO, is well beyond the scope of this series. However, I must stop to point out that a diet low in simple sugars and high-GI simple starches, and that eliminates the antinutrients, enzyme inhibitors, and gut irritants found in grains and (to a lesser extent) beans and many nuts, is beneficial for almost all such issues.
Yes, I’m talking about a functional paleo diet.
(Those interested in digging more deeply into the subject might want to watch Dr. BG’s presentation at AHS 2011. Here’s the video, and here are the slides.)
Satiety Failure #3: Energy Stuck In Storage
We use energy continually throughout the day. And depending on how active we are, our energy usage can go from ‘minimal’ (sitting on couch watching TV) to ‘moderate’ (walking, intense mental activity) to ‘huge’ (sprinting at top speed, lifting heavy objects).
Yet we do not eat a constant stream of calories that corresponds exactly to our current degree of physical and mental effort. Our bodies must store the energy we eat for later usage. And as our storage capacity for glucose (as glycogen) is very limited, our body’s long-term energy storage is…fat.
Glycogen: A Short Explanation
 A glycogen hairball.
Glycogen is a big hairball of glucose molecules connected to a protein called glycogenin, and it’s how our body prefers to store glucose. However, our body’s glycogen reserves are small: perhaps 70g in the liver and 200g in all our skeletal muscles, combined.* (The second capacity increases with muscle mass and training: a large, muscular, trained athlete can store perhaps 400g.) Furthermore, glycogen cannot be shuttled out of or between muscles: it’s only available to the muscle containing it.**
This isn’t very much energy: about 1100 calories’ worth, of which only 240 are available to the brain via the liver. So our bodies store most excess energy as fat, which is more energy-dense (approximately 9 calories/gram vs. 4), and for which we have a basically infinite storage capacity in our adipose tissue (‘fat cells’).
* Figures cited for muscle glycogen storage vary widely, and I haven’t found an authoritative source. Furthermore, it’s not clear how deeply storage is or can be depleted by exercise: even running to exhaustion only depletes specific muscles by perhaps 40-60%.
** This study (hat tip to alert commenter Franco) appears to show that glycogen can move slowly between muscles (over the course of hours), but only after exercise and only when fasted. Transfer doesn’t appear to be significant during exercise.
But what if we had trouble using fat for energy—or using energy at all? Clearly we’d have a problem: we would eat food, and as soon as the energy was either used or stored, we’d be hungry again—even though we were gaining weight!
This is exactly what happens to many people.
I’ve previously discussed metabolic flexibility and the RER (“Respiratory Exchange Ratio”), also known as the RQ (“Respiratory Quotient”), at length in this article. Metabolic flexibility (“met flex”) is the ability of our cells (specifically, our mitochondria) to switch back and forth between glucose oxidation and fat oxidation for energy, and the RER/RQ is how we measure what proportion of glucose vs. fat we’re burning.
It turns out that:
- The obese have impaired metabolic flexibility.
- The obese have impaired mitochondrial capacity to turn nutrients into energy in the muscles.
- The obese have an impaired ability to oxidize fat for energy, which we can objectively measure.
- Both the formerly obese and the soon-to-be-obese also suffer these impairments.
Linda Bakkman, Maria Fernström, Peter Loogna, Olav Rooyackers, Lena Brandt, Ylva Trolle Lagerros
Reduced Respiratory Capacity in Muscle Mitochondria of Obese Subjects
Obes Facts 2010;3:371-375
(fulltext available as PDF)
“Obese subjects had a decreased respiratory capacity per mitochondrial volume compared to the reference groups: this was evident in state 4 (65% and 35% of reference group A and B, respectively) and state 3 (53% and 29% of A and B, respectively) (p < 0.05)."
In other words, obese people have a greatly decreased ability to create energy from the nutrients they ingest.
The ability to oxidize fat is also impaired. How great is this impairment?
Ranneries, C., Bulow, J., Buemann, B., Christensen, N. J.,
Madsen, J., & Astrup, A.
Fat metabolism in formerly obese women.
AJP – Endo January 1998 vol. 274 no. 1 E155-E161
“…Fat mobilization both at rest and during exercise is intact in FO [formerly obese], whereas fat oxidation is subnormal despite higher circulation NEFA levels. The lower resting EE [energy expenditure] and the failure to use fat as fuel contribute to a positive fat balance and weight gain in FO subjects.”
The difference is remarkable. From Table 2 of Ranneries et.al., we find these startling facts:
- Normal subjects are burning 30% more calories at rest than the formerly obese.
- Normal subjects are burning 7% carbs and 78% fat at rest, whereas formerly obese subjects are burning 49% carbs and 34% fat at rest!
Let that sink in for a moment. These aren’t even the obese: they’re the formerly obese. So the theory that some people become “metabolically broken” has factual support.
Here’s the graph of fat oxidation before, during, and after an hour-long bout of exercise. The triangles are controls, the circles are the formerly obese:
 Fraction of energy expenditure covered by fat oxidation (E%) during rest (t = 0 min), exercise (t = 0–60 min), and recovery (t = 75 min) in formerly obese subjects (FO, •) and matched controls (C, ▿). Values are means ± SD.
We can easily see that normal subjects have metabolic flexibility—the ability to switch back and forth between carb and fat oxidation—whereas the formerly obese are impaired. (Though exercise does increase metabolic flexibility, as I’ve previously noted.)
Continuing, we see that RER (= RQ) is predictive of future obesity:
F. Zurlo, S. Lillioja, A. Esposito-Del Puente, B. L. Nyomba, I. Raz, M. F. Saad, B. A. Swinburn, W. C. Knowler, C. Bogardus, and E. Ravussin
Low ratio of fat to carbohydrate oxidation as predictor of weight gain: study of 24-h RQ
AJP – Endo November 1990 vol. 259 no. 5 E650-E657
“Subjects with higher 24-h RQ (90th percentile) independent of 24-h energy expenditure were at 2.5 times higher risk of gaining greater than or equal to 5 kg body weight than those with lower 24-h RQ (10th percentile).”
There are many more interesting papers I could cite and quote here—but if I do so, this article will expand to an unreadable size! So, instead of bombarding you with more citations, I’ll quote this excellent research review, which contains more citations for the above facts, and even more fascinating data for which space does not permit discussion.
Mary Madeline Rogge
The Role of Impaired Mitochondrial Lipid Oxidation in Obesity
Biol Res Nurs April 2009 vol. 10 no. 4 356-373
(fulltext available as PDF)
“Figure 2. In obesity, impaired glucose tolerance, and type 2 diabetes, mitochondrial beta-oxidation is decreased in skeletal muscle cells.”
[Beta-oxidation is the process by which mitochondria produce energy from fat.]
“Carnitine palmitoyltransferase 1 (CPT1) activity, necessary for the transport of long-chain fatty acids into the cell, is diminished, leading to the accumulation of fatty acyl-CoA within the cytosol. Under the influence of the enzyme acetyl-CoA carboxylase (ACC), unmetabolized fatty acyl-CoA is converted to malonyl-CoA and committed to the re-synthesis of fatty acids, which can accumulate within the cell or be transported to other tissues as triglycerides. The reduced ability to use fatty acids for ATP production increases obese individuals’ reliance on glycolysis and decreases their exercise capacity.”
If you want to learn more, p. 361 of the full text and the subsection “Decreased Fat Oxidation” will be quite illuminating, and I strongly recommend reading it. For that matter, just read the whole paper, as it’s an excellent overview and summary.
These facts provide an explanation for the additional fact that some people, particularly the obese, do not find carbohydrate to be satiating:
Chambers L, Yeomans MR.
Individual differences in satiety response to carbohydrate and fat. Predictions from the Three Factor Eating Questionnaire (TFEQ).
Appetite. 2011 Apr;56(2):316-23. Epub 2011 Jan 8.
“Those scoring high on the TFEQ-disinhibition scale consumed more energy at the snack test than those with low TFEQ-disinhibition, but this was only following the high carbohydrate breakfast. … In normal-weight females the tendency to overeat may be related to insensitivity to the satiating effects of carbohydrate.”
An impaired ability to burn fat for energy means that you will no longer be sated once your blood sugar drops, leaving you hungry again—even though most of the energy has been stored and you are in positive energy balance. In other words, the combination of impaired fat oxidation and a high-carbohydrate, low-fat diet is likely to leave you both hungry and gaining weight. (See this study for a real-world instrumented comparison.)
Impaired fat oxidation also causes the “low carb flu”. You’re forcing your body to adapt to burning fat by refusing to provide it with carbohydrate—but since your mitochondria don’t burn fat very well, you’ll have very little energy until you adapt.
I conclude this section with several thoughts:
First, this is not the “greedy fat cells” theory of obesity, which posits an inability of the obese to retrieve fat from fat cells into circulation. That ability appears to be intact. What is indisputably damaged is the mitochondrial function of the obese, the formerly obese, and the soon-to-be-obese, and their ability to oxidize fat for energy.
Second, any valid theory of obesity or its treatment must take the facts of these metabolic impairments into account.
Third, satiety is indeed a primary driver of hunger, and without satiety we will always be hungry—but as important as it is, this is only one part of the answer to “Why are we hungry?”
Conclusion
A lack of satiety will leave us hungry no matter what else we do to compensate.
We fail to achieve satiety in the following ways:
- By not ingesting the energy and/or nutrients our body requires.
- By not absorbing the energy and/or nutrients our body requires.
- By an inability to retrieve the energy and/or nutrients our bodies have stored, due to impaired metabolic flexibility caused by impaired mitochondrial function and, most importantly, impaired fat oxidation.
Thank you for reading all the way through this long but (I believe) rewarding article! The following installments explore failures of the other hunger drives—and once we understand the failures, we can finally begin to construct workable solutions.
Live in freedom, live in beauty.
JS
Continue to Part V: When Satiation Fails…Calorie Density, Oral Processing Time, and Rice Cakes vs. Prime Rib.
This is Part IV of an ongoing series. Go back to Part I, Part II, or Part III.
Did you find this article interesting or illuminating? I certainly hope so, because writing it was an ordeal. Use the buttons below to share it!
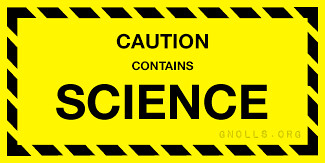
Important note! For a more up-to-date exploration of this subject, I strongly recommend my 2013 AHS presentation “What Is Metabolic Flexibility, And Why Is It Important?”
Most of us who eat a low-carbohydrate diet—Paleo, Primal, Atkins, or otherwise—experience anywhere from a couple days to a couple weeks of low energy as we adjust to it, an experience known informally as the “low carb flu”. And some people never seem to adjust.
Here’s why—and here are some ideas that might help you if you’re having trouble adjusting!
Note that low-carb isn’t an objective of a paleo diet: it’s just the usual consequence of eliminating grains and sugars.
It’s certainly possible to eat a higher-carb paleo diet—and it’s a good idea if you’re doing frequent, intense workouts like HIIT, Crossfit, or team sports after school—but you’d have to eat a lot of potatoes and bananas to get anywhere near the same amount of carbohydrate you used to get from bread, pasta, cereal, and soda.
Burning Food For Energy: Glycolysis and Beta-Oxidation
Our bodies have several ways to turn stored or ingested energy into the metabolic energy required to move around and stay alive. This is called cellular respiration.
The two main types of cellular respiration are anaerobic (which does not require oxygen) and aerobic (which requires oxygen). Anaerobic metabolism, also known as fermentation, is nineteen times less efficient—and we can only maintain it for short periods, because its waste products build up very quickly. This is why we can’t sprint for long distances.
We spend most of our time in aerobic metabolism. Our two primary aerobic sources of energy are glycolysis, which converts glucose to energy, and beta-oxidation, which converts fat to energy.
A Short Metabolic Digression Explaining The Above (Optional)
Strictly speaking, glycolysis is the start of both the aerobic and anaerobic oxidation of glucose: it converts glucose to pyruvate.
In the aerobic oxidation of glucose, the pyruvate is transported into the mitochondria, whereupon it is converted to acetyl-CoA and fed into the TCA cycle (aka the citric acid cycle or Krebs cycle, depending on how long ago you took your biology course.) This produces 19 times more energy than the original glycolysis!
In the anaerobic oxidation of glucose (“lactic acid fermentation”), the pyruvate is instead converted to lactic acid, which produces no more energy.
In the aerobic oxidation of fat (“beta-oxidation”), the fat is transported into the mitochondria, whereupon it is sliced up into individual acetyl-CoA molecules, each of which enters the TCA cycle.
Humans have no way to anaerobically oxidize fat.
 A graphic flowchart of glycolysis. You know it's SCIENCE! because there are a lot of molecules and arrows.  And here's a flowchart of beta-oxidation.
Glucose is the simple sugar all cells use for glycolysis, and it’s the most common. The other simple sugars we can digest are galactose (found primarily in milk), which we convert to glucose—and fructose (found primarily in fruit, table sugar, corn syrup, and honey), which our liver converts directly to glycogen or fat.
Starch is just a bunch of glucose molecules stuck together. In fact, “complex carbohydrates” in general are just sugars stuck together…and we can only absorb them through the intestine once they are broken down into individual simple sugars by our digestive system. In other words, all “carbohydrates” are just sugar.
There is a lot more to talk about here, including glycogen storage and retrieval, gluconeogenesis, and de novo lipogenesis…but explaining all the pathways of digestion, energy storage, and cellular respiration would be an entire book in itself!
Moving on: while it’s OK for fat to hang around in our bloodstream for a while, high blood sugar is actively toxic to our tissues. (The long-term consequences of untreated diabetes—heart, kidney, nerve, eye, and muscle damage, leading to numbness, blindness, amputations, strokes, and death—are basically just long-term glucose poisoning.) So after we eat something containing any amount of carbohydrate, insulin ensures that the glucose is immediately taken into our cells and either burned for energy, stored, or converted into palmitic acid—a saturated fat!
This is why a “low-fat, high-carb” diet is really a high-fat diet. Unless your “high-carb” diet involves an intravenous glucose drip carefully metered to keep your blood sugar constant, most of the ‘carbohydrates’ (sugars) you eat will be converted either to glycogen or to palmitic acid (again, a saturated fat) before you use them. “Soluble fiber” and other indigestible carbohydrates are fermented into short-chain saturated fats, like butyric acid, in your colon. Fructose, of course, is converted directly to liver glycogen or to palmitic acid. And if you’re losing weight by burning your own fat, keep in mind that human fat has roughly the same composition as lard—approximately 40% saturated!
You might ask yourself if it makes sense that natural selection would select us to store energy in the form of something directly harmful to us. If saturated fat is really so terrible, and polyunsaturated fat is really so healthy, why doesn’t our body store energy as linoleic acid, like grains do?
Metabolic Flexibility and the Respiratory Exchange Ratio
If we have excess glucose in our bloodstream, our muscles will burn it first, because it’s toxic. But eventually we run out of glucose, and that’s when our bodies need to switch over to beta-oxidation—burning fat. The ability to switch back and forth between the two processes is called “metabolic flexibility” in the scientific literature.
Metabolic flexibility varies dramatically from individual to individual, which we would expect based on the widely varying experiences people report with low-carb diets. So how do scientists figure out what fuel our bodies are burning?
It turns out that beta-oxidation (fat-burning) produces less carbon dioxide than glycolysis (sugar-burning)—and we can measure that in our breath. The ratio of CO2 to O2 is 0.7 for beta-oxidation and 1.0 for glycolysis…so an RER (Respiratory Exchange Ratio) of 0.7 indicates pure fat-burning, and 1.0 and above indicates pure sugar burning. (You can read more about the RER here.)
Typical healthy people have a resting, fasting RER of approximately 0.8. Therefore, we can easily see that the frequent vegetarian and vegan claims of “Nothing else can provide any energy without first being converted to carbs” and “You can get plenty of energy from fat, but you have to go into ketosis to do it” are—like most nutritional claims made by veg*ans—complete bunk.
Metabolic Flexibility: The “Low Carb Flu” Is Not Your Imagination
It shouldn’t be a surprise that the obese and diabetic tend to have higher resting RERs, and that higher RER is a significant predictor of future obesity. If our ability to burn fat for energy is impaired, we’re going to have a hard time losing weight, and we’ll become ravenously hungry when our blood sugar runs out no matter how much fat we have available to burn.
Is this sounding familiar to anyone?
Sounds like the “low carb flu”, doesn’t it? When we talk about our metabolic “set point”, part of what we’re talking about is metabolic flexibility. It does no good to have a huge store of fat if we can’t burn it for energy!
Int J Obes Relat Metab Disord. 1992 Sep;16(9):667-74.
Fasting respiratory exchange ratio and resting metabolic rate as predictors of weight gain: the Baltimore Longitudinal Study on Aging.
Seidell JC, Muller DC, Sorkin JD, Andres R.
“…The adjusted relative risk of gaining 5 kg or more in initially non-obese men with a fasting RER of 0.85 or more was calculated to be 2.42 (95% confidence interval: 1.10-5.32) compared to men with a fasting RER less than 0.76.”
Furthermore, it turns out that people with a family history of type II diabetes, but who don’t yet have it themselves, have higher RERs and impaired metabolic flexibility.
Diabetes August 2007 vol. 56 no. 8 2046-2053
Impaired Fat Oxidation After a Single High-Fat Meal in Insulin-Sensitive Nondiabetic Individuals With a Family History of Type 2 Diabetes
Leonie K. Heilbronn1, Søren Gregersen2, Deepali Shirkhedkar1, Dachun Hu1, Lesley V. Campbell1
“…An impaired ability to increase fatty acid oxidation precedes the development of insulin resistance in genetically susceptible individuals.”
Also see:
AJP – Endo November 1990 vol. 259 no. 5 E650-E657
Low ratio of fat to carbohydrate oxidation as predictor of weight gain: study of 24-h RQ
F. Zurlo, S. Lillioja, A. Esposito-Del Puente, B. L. Nyomba, I. Raz, M. F. Saad, B. A. Swinburn, W. C. Knowler, C. Bogardus, and E. Ravussin
This is very important: we can see that impaired fat oxidation must be related to the causes, not the consequences, of obesity and diabetes. So we’ve struck another blow to “calories in, calories out”, and the idea that you’re fat just because you’re lazy.
In support of this theory, I note this paper, which contains the following graph of several individuals’ RER in response to a high-fat diet vs. a moderate-fat diet. Keep in mind that the change was only from 37% to 50% fat, which is relatively minor, and the paper doesn’t tell us what fats were being consumed…but this graph is still instructive:

The top graph is the average, the bottom graph is for each individual. Note that some adapted right away, some took several days, and some were still not adapted on day 4!
Higher RER isn’t all bad. It’s associated with having more fast-twitch muscle fibers, which are associated with a greater ability to build muscle mass. This fits the anecdotal evidence that people who gain fat easily also tend to gain muscle easily, whereas skinny people have a much harder time bulking up.
So maybe you’re lucky, or already in good health, and you adapt relatively quickly to a low-carb diet. But what if you’re not? What if you’re stuck with the “low carb flu”?
Regaining Your Metabolic Flexibility
Obviously we’d like to regain our metabolic flexibility. But how? Here’s one way:
Journal of Applied Physiology September 2008 vol. 105 no. 3 825-831
Separate and combined effects of exercise training and weight loss on exercise efficiency and substrate oxidation
Francesca Amati,1 John J. Dubé,2 Chris Shay,3 and Bret H. Goodpaster1,2
“…Exercise training, either alone or in combination with weight loss, increases both exercise efficiency and the utilization of fat during moderate physical activity in previously sedentary, obese older adults. Weight loss alone, however, significantly improves neither efficiency nor utilization of fat during exercise.”
Diabetes September 2003 vol. 52 no. 9 2191-2197
Enhanced Fat Oxidation Through Physical Activity Is Associated With Improvements in Insulin Sensitivity in Obesity
Bret H. Goodpaster, Andreas Katsiaras and David E. Kelley
“Rates of fat oxidation following an overnight fast increased (1.16 ± 0.06 to 1.36 ± 0.05 mg · min−1 · kg FFM−1; P < 0.05), and the proportion of energy derived from fat increased from 38 to 52%."
October 15, 2009 The Journal of Physiology, 587, 4949-4961.
Improved insulin sensitivity after weight loss and exercise training is mediated by a reduction in plasma fatty acid mobilization, not enhanced oxidative capacity
Simon Schenk1, Matthew P. Harber1, Cara R. Shrivastava1, Charles F. Burant1,2 and Jeffrey F. Horowitz1
“…Resting fatty acid oxidation was unchanged after the intervention in WL [weight loss]. Consistent with an increase in maximal oxidative capacity, resting whole-body fatty acid oxidation was increased more than 20% after WL + EX [weight loss + exercise].”
In other words, despite the title, weight loss plus exercise increased resting fat oxidation…but just losing weight did not!
AJP – Endo April 2008 vol. 294 no. 4 E726-E732
Skeletal muscle lipid oxidation and obesity: influence of weight loss and exercise
Jason R. Berggren,1,2 Kristen E. Boyle,1,2 William H. Chapman,4 and Joseph A. Houmard1,2,3
“10 consecutive days of exercise training increased (P ≤ 0.05) FAO [fatty acid oxidation] in the skeletal muscle of lean (+1.7-fold), obese (+1.8-fold), and previously extremely obese subjects after weight loss (+2.6-fold)…These data indicate that a defect in the ability to oxidize lipid in skeletal muscle is evident with obesity, which is corrected with exercise training but persists after weight loss.”
How about that? It turns out that exercise is important after all…not because of the calories you burn by exercising, which you usually replace right away because you’re hungry, but because it helps you regain metabolic flexibility. Exercise stimulates your body to burn more fat, both during exercise and at rest.
And that’s what health is about: we’re not interested in losing weight if it just means losing muscle. We’re interested in losing fat.
There are other benefits beyond fat loss, too: exercise tends to normalize broken metabolisms.
Diabetes March 2010 vol. 59 no. 3 572-579
Restoration of Muscle Mitochondrial Function and Metabolic Flexibility in Type 2 Diabetes by Exercise Training Is Paralleled by Increased Myocellular Fat Storage and Improved Insulin Sensitivity
Ruth C.R. Meex1, Vera B. Schrauwen-Hinderling2,3, Esther Moonen-Kornips1,2, Gert Schaart1, Marco Mensink4, Esther Phielix2, Tineke van de Weijer2, Jean-Pierre Sels5, Patrick Schrauwen2 and Matthijs K.C. Hesselink1
“Mitochondrial function was lower in type 2 diabetic compared with control subjects (P = 0.03), improved by training in control subjects (28% increase; P = 0.02), and restored to control values in type 2 diabetic subjects (48% increase; P < 0.01). Insulin sensitivity tended to improve in control subjects (delta Rd 8% increase; P = 0.08) and improved significantly in type 2 diabetic subjects (delta Rd 63% increase; P < 0.01). Suppression of insulin-stimulated endogenous glucose production improved in both groups (−64%; P < 0.01 in control subjects and −52% in diabetic subjects; P < 0.01). After training, metabolic flexibility in type 2 diabetic subjects was restored (delta respiratory exchange ratio 63% increase; P = 0.01) but was unchanged in control subjects (delta respiratory exchange ratio 7% increase; P = 0.22).”
Did you catch that? “Metabolic flexibility in type 2 diabetic subjects was restored”?
Unfortunately, this study didn’t measure resting fat oxidation, like the others—but it does suggest that there’s no need to kill yourself with “Biggest Loser”-style misery. 30 minutes of cycling at 55% of maximum effort twice a week, and one session of weight training once a week, was enough to restore metabolic flexibility. That doesn’t sound very intimidating, does it? (And there are many better and more entertaining ways to get half an hour of moderate aerobic exercise than sitting on a stationary bike.)
“Aerobic exercise was carried out on a cycling ergometer twice a week for 30 min at 55% of a previously determined maximal work load (Wmax). Resistance exercise was performed once a week and comprised one series of eight repetitions at 55% of subjects’ previously determined maximal voluntary contraction (MVC) and two series of eight repetitions at 75% MVC and focused on large muscle groups (Chest press, leg extension, lat pull down, leg press, triceps curls, biceps curls, abdominal crunches, and horizontal row).”
…Yet You Must Take Advantage Of Your Newfound Metabolic Flexibility
Of course, our newly-regained flexibility won’t help if we stuff ourselves with the government-recommended 7-11 servings of “heart-healthy whole grains” (= “carbs”, = sugar) per day, because we will be constantly burning sugar. Only when we’re done burning glucose can we use our newfound flexibility to burn some fat.
That’s one reason, among many, why I eat a paleo diet—and why I don’t snack. (For more on that subject, read “Why Snacking Makes You Weak, Not Just Fat”.)
A Short Digression: Please Stay Off The “Faileo Diet”
Some ‘paleo’ books still insist that saturated fat is bad for you and paleolithic people didn’t eat much of it, which is absolute nonsense. But your calories have to come from somewhere…if not fat, then from protein or carbohydrates. And since those same books also usually disallow potatoes and other convenient sources of starch, you’re basically stuck eating lots of lean protein.
As a result, you’ll eat very few calories, because of the satiating effect of protein—which is fine if you’re just trying to lose weight, but disastrous if you’re physically active, because you’ll be perpetually exhausted. This is why fat-phobic ‘paleo’ is sometimes called the “Faileo Diet”.
The Difference Between Beta-Oxidation and Ketosis
Here’s where I say something that might be controversial: I think going cold-turkey VLC (very low carb) or zero-carb makes the transition much harder, particularly for people who are already physically active.
Beta-oxidation (fat-burning) occurs nearly continually, and produces much of our energy at rest once insulin has cleared any sugar spike out of our system. However, our body does have some requirement for glucose, which it satisfies in the short-term primarily by having the liver make it—a process called gluconeogenesis.
If we eat zero carbs, or very few, over a period of time, our body enters a state called ketosis, in which some of our tissues that used to require glucose shift over to burning ketone bodies, which are alternative products of fat metabolism. And while it is true that our brains and hearts actually run more efficiently on ketones, it takes several weeks for our bodies to fully adapt. Meanwhile, we lack energy for high-effort activities, because our muscles are depleted of glycogen, which is made from glucose.
So you might not have the “low-carb flu”—you might be stuck in an unnecessary multi-week rut of keto-adaptation.
Interested in learning more about ketosis? Read Stephen Phinney’s “Ketogenic Diets and Physical Performance” (Nut Metab 2004, 1:2) for more information about ketosis and the process of keto-adaptation. Once you’ve read that, if you’re deeply interested in the ketotic state, try ketotic.org…and if you’re interested in a ketogenic diet that isn’t nutrient-deficient or disgusting, try these articles from the Drs. Jaminet (Ketogenic Diets I, Ketogenic Diets II).
There is a persistent myth that ketosis is dangerous: it’s not. People (including some doctors) commonly confuse it with ketoacidosis, a pathological state usually only found in uncontrolled diabetics and (rarely) raging alcoholics.
Even worse, you might be stuck in the state informally known as “Low Carb Limbo”—in which you’re eating too few carbohydrates to fuel high-effort, glycolytic activity, but too many carbohydrates to ever keto-adapt.
If you’re active and determined to keto-adapt, read this excellent article from Primal North, “Keto-Adaptation vs. Low Carb Limbo”.
Conclusion: Stay Out Of The Muddy Middle
In summary, it’s much easier and quicker to burn fat via beta-oxidation than it is to adapt to ketosis…so unless ketosis is your goal, you might be making your transition to a healthy diet much harder by keeping your carb intake too low.
I think that if we keep our carbohydrate intake near our body’s requirement while not in ketosis, which is perhaps 15-20% of total calories—and only eat those carbohydrates with meals involving complete protein and fat, not by themselves—most of us should be able to gain the fat-burning benefits of metabolic flexibility without suffering the pain of trying to adapt to ketosis. So if you’re new to Paleo or low-carb eating, you’re stuck with long-term “low-carb flu”, and especially if you’re already physically active, try adding some root starches like potatoes or sweet potatoes to your meals. (Or white rice, if you’re following the Perfect Health Diet.)
(And if you’re determined to keto-adapt, go fully ketogenic, as per “Keto-Adaptation vs. Low Carb Limbo.”)
Live in freedom, live in beauty.
JS
For more information, continue reading my 2013 AHS presentation “What Is Metabolic Flexibility, And Why Is It Important?”
Was this article helpful to you? Please share it using the buttons below.
|
“Funny, provocative, entertaining, fun, insightful.”
“Compare it to the great works of anthropologists Jane Goodall and Jared Diamond to see its true importance.”
“Like an epiphany from a deep meditative experience.”
“An easy and fun read...difficult to put down...This book will make you think, question, think more, and question again.”
“One of the most joyous books ever...So full of energy, vigor, and fun writing that I was completely lost in the entertainment of it all.”
“The short review is this - Just read it.”
Still not convinced?
Read the first 20 pages,
or more glowing reviews.
Support gnolls.org by making your Amazon.com purchases through this affiliate link:

It costs you nothing, and I get a small spiff. Thanks! -JS
.
Subscribe to Posts
|
Gnolls In Your Inbox!
Sign up for the sporadic yet informative gnolls.org newsletter. Since I don't update every day, this is a great way to keep abreast of important content. (Your email will not be sold or shared.)
IMPORTANT! If you do not receive a confirmation email, check your spam folder.
|